Journal of Cancer Research & Therapy
An International Peer-Reviewed Open Access Journal
ISSN 2052-4994


- Download PDF
- |
- Download Citation
- |
- Email a Colleague
- |
- Share:
-
- Tweet
-
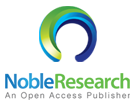
Journal of Cancer Research & Therapy
Volume 2, Issue 9, September 2014, Pages 134–143
Original researchOpen Access
Plant-based omega-3 stearidonic acid enhances antitumor activity of doxorubicin in human prostate cancer cell lines
-
Caitlin Hellmich Trebelhorn1

- 1 Department of Anatomy, Physiology, and Pharmacology, College of Veterinary Medicine, Auburn University, Auburn, AL, USA
- 2 Department of Biomedical Sciences, College of Veterinary Medicine, Tuskegee University, Tuskegee, AL, USA
*Corresponding author: Mahmoud Mansour, Department of Anatomy, Physiology, and Pharmacology, College of Veterinary Medicine, Auburn University, Auburn, AL 36849, USA. Tel.: 334-844-6741; Fax: 334-844-4542. E-mail: mansoma@auburn.edu
Received 24 May 2014 Revised 25 July 2014 Accepted 8 August 2014 Published 15 August 2014
DOI: http://dx.doi.org/10.14312/2052-4994.2014-21
Copyright: © 2014 Trebelhorn CH, et al. This is an open-access article distributed under the terms of the Creative Commons Attribution License, which permits unrestricted use, distribution and reproduction in any medium, provided the original author and source are credited.
AbstractTop
Doxorubicin (DOX) is a first choice cytostatic drug in treatment of many cancers but among its side effects is cardiac toxicity. Stearidonic omega-3 fatty acid (SDA) has cardiac protective qualities and, therefore, we investigated the combinatory effects of DOX and SDA on proliferation/viability in the human prostate cancer (PCa) cell lines LNCaP, PC3, and DU145 as well as possible modulatory effects on expression of androgen receptor (AR), peroxisome proliferator-activated receptor gamma (PPARγ) and nuclear factor kappa-light-chain-enhancer of activated B cells (NF-κB) nuclear transcription factors implicated in tumorigenesis. The median inhibitory effects (IC50) of SDA were 601, 116, 145 µM and that of DOX were 802, 761, 363 nM in LNCaP, PC3 and DU145, respectively. Equipotent combinations of DOX and SDA based on 2-fold dilutions and constant combination ratios derived from the above IC50 values suggested anticancer synergism with a combination index (CI) of less than 1 as determined using the Chou-Talalay method based on the median-effect equation and the mass action law. Stearidonic acid strongly inhibited TNFα-activated NF-κB in stably transduced LNCaP cells. We used immunocytochemistry, real-time PCR, western blotting, and transactivation assays to demonstrate inhibition of agonist-activated AR and PPARγ expression following treatment with DOX and SDA singly or in combination. This study provides proof-of-concept for using DOX and SDA in combination to reduce dose and toxicity of DOX in PCa clinical translation studies.
Keywords: stearidonic acid; doxorubicin; drug combination; peroxisome proliferator-activated receptor; nuclear factor kappa-light-chain-enhancer; antitumor activity; human prostate cancer
IntroductionTop
Prostate cancer is one of the most frequently diagnosed cancers in American men [1] in whom age, ethnicity, and a positive family history are the most important risk factors [2]. Therapeutic options for treatment of castration-resistant prostate cancer (CRPC) at the late stage (stage IV) remain limited despite intense efforts aimed at finding an effective cure [3, 4]. Combination therapy in CRPC offers an opportunity to maximize therapeutic efficacy.
Drug combination therapy is actively pursued in CRPC to achieve the following: i) reduce dose and toxicity; ii) achieve synergism to maximize therapeutic efficacy; and iii) minimize drug resistance [5]. For example, docetaxel (taxotere) is used in combination with estramustine [6] or the steroid drug prednisone [7]. Other combinations include cabazitaxel (jevtana) with the steroid prednisone in patients with docetaxel-resistant prostate cancer [8].
Fish-based omega-3 (n-3) fatty acids were reported to increase the efficacy of several chemotherapeutic drugs in various cancers. For example, n-3 fatty acids increased the efficacies of doxorubin [9, 10], epirubicin [11], mitomycin C [12], arabinosylcytosine [13], and CPT-11 [14] in breast, lung, and lymphocytic cancers when included in the diet of tumor-bearing rodents or supplemented in cell culture medium.
Doxorubicin (DOX), also called adriamycin, is an anthracycline antibiotic used as a chemotherapeutic drug in treatment of several cancers including breast, lung, gastric, ovarian, thyroid, non-Hodgkin’s and Hodgkin’s lymphoma, multiple myeloma, sarcoma, and pediatric cancers [15-17]. DOX disrupts topoisomerase-II-mediated DNA repair and generates free radicals that damage cancer cell membranes, DNA, and proteins [18], with cardiac toxicity remaining a major side effect [19]. It is of interest, however, to note previously reported synergy between DOX and other drugs such as suramin [20], docetaxel [21] and sildenafil (Viagra) [22] in treatment of prostate cancer. The latter drug is reported to ameliorate cardiac dysfunction associated with DOX therapy.
Stearidonic acid [SDA; 18:4 (n-3)] is an eicosapentaenoic acid (EPA) and docosahexaenoic acid (DHA) precursor that, like DOX, lowers the proliferation index and increases apoptosis in xenograft prostate cancer models [23, 24]. Additionally, SDA increases EPA accumulation in the heart and consequently is considered as a “pro-EPA” omega-3 fatty acid that, like EPA and DHA [25, 26], has cardiovascular protective benefits and lipid lowering effects [27, 28]. Ameliorating the cardiac toxicity of DOX by combination with SDA could be exploited in the treatment of HRPC.
The antitumor mechanism of SDA in prostate cancer is unclear. Studies indicate antitumor and health-promoting effects of (n-3) long-chain polyunsaturated fatty acids (LCPUFA) from fish [24, 29]. For example, treatment of prostate cancer cells with EPA and DHA induces cell cycle arrest and apoptosis [30] and decreases prostate tumor growth in vivo [31]. Other molecular mechanisms of EPA and DHA include modulation of the composition of cell membrane phospholipids and inhibition of pathways involved in growth promoting prostaglandins by competing with arachidonic acid for Cox-2 [32]. In addition, EPA and DHA modulate transcription nuclear factors such as androgen receptor (AR) [33], nuclear factor kappa-light-chain-enhancer of activated B cells (NF-κB) and peroxisome proliferator-activated receptor-γ (PPARγ) [34].
Given that several human prostate and other cancer cell lines exhibit resistance to DOX [35, 36] and that DOX in combination with other drugs has synergistic effects in prostate cancer treatment [20-22], we wanted to examine the effects of DOX in combination with SDA and to provide proof-of-concept for enhanced antitumor activity of DOX/SDA. We describe observations that suggest that the sum of antitumor effects of DOX/ SDA is superior to DOX or SDA when used alone.
Materials and methodsTop
Cells/ Reagents
Normal human prostate epithelial cells, RWPE-1, human prostate adenocarcinoma cell lines, PC3, DU145, and LNCaP, 3-(4,5-dimethylthiazol-2-yl)-2-5-diphnyltetrazolium bromide (MTT) cell viability kits, and HepG2 human liver carcinoma were obtained from the American Type Culture Collection (Manassas, VA). RPMI-1640 with HEPES and L-Glutamine (with and without phenol red) and phosphate buffered saline (PBS) without calcium and magnesium were purchased from Lonza (Walkersville, MD). Keratinocyte-SFM media for propagation of RWPE-1 was purchased from Gibco Life Technologies (Carlsbad, CA). Advantage and charcoal-treated fetal calf serums (FCS) were purchased from Atlanta Biologicals (Lawrenceville, GA). Rosiglitazone and troglitazone (PPARγ agonists), SDA, EPA and DHA omega-3 fatty acids were obtained from Cayman Chemical (Ann Arbor, MI). Trypsin-EDTA, penicillin-streptomycin antibiotics, and cell culture supplies were purchased from VWR International, LCC (Atlanta, GA). Testosterone, DMSO, doxorubicin and TNFα were purchased from Sigma (St Louis, MO). Validated RT-PCR primers for AR and PPARγ were obtained from SABiosciences/Qiagen (Germantown, MD). TRIzol reagent was purchased from Invitrogen-Life Technologies Inc. (Grand Island, NY).
Primary antibodies, AR (Sc-816) and PPARγ (Sc-7273) were obtained from Santa Cruz Biotechnology, Inc. (Dallas, TX). DyLight 488-conjugated goat anti-rabbit IgG (Thermo Scientific, Rockford, IL) and Alexa Flour 568-conjugated goat anti-mouse IgG (Invitrogen-Life Technologies, Grand Island, NY) were used to visualize primary binding in immunocytochemistry assays.
Cells maintenance
LNCaP, PC3, and DU145 human PCa cells were maintained in RPMI-1640 with HEPES and L-Glutamine media supplemented with 10% (v/v) FCS and 1% (v/v) streptomycin-penicillin antibiotics (Life Technologies, Grand Island, NY). RWPE-1 cells were maintained in keratinocyte-SFM media containing bovine pituitary extract and human recombinant epidermal growth factor. HepG2 cells were grown in Dulbecco’s modified Eagle’s medium (DMEM; Life Technologies) supplemented with 10% FCS and 100 U/ml penicillin and 100 µg/ml streptomycin as described previously [37]. Cells were grown at 37˚C under a 5% CO2, humidified atmosphere in T-75 vented-cap tissue culture flasks until 75-90% confluency was achieved. For cell cultures, PCa cells were treated with 0.25% Trypsin and counted using a TC-10 automated cell counter (Bio-Rad, Hercules, CA).
MTT cell proliferation assays
MTT assay protocol
Cells in logarithmic-phase growth were collected via trypsinization and counted using a TC-10 automated cell counter. The effect of DOX and SDA on cell viability and /or proliferation was assessed by MTT cell proliferation assay kit according to the manufacturer’s instructions. Color absorbance (OD) was determined at 570 nm using SpectraMax-Plus-384 microplate reader (Molecular Devices, Sunnyvale, CA). Experiments were repeated at least three times.
Drug combination
DOX or polyunsaturated fatty acids were dissolved in ethanol or DMSO and further diluted in charcoal-treated, phenol red free RPMI-1640 media to desired concentrations. Drug mixtures for calculation of combination index (CI) values were based on the median effect analysis method [5]. Two-fold serial dilutions of working concentrations were prepared in charcoal-treated RPMI-1640 with five concentrations above and below the calculated IC50 for each drug in each cell line. SDA/DOX were mixed using constant ratios corresponding to 0.03, 0.06, 0.12, 0.25, 0.5, 1, 2, 4, 8, 16, 32 times the IC50 for each drug in each cell line. Next, the culture media was suctioned from the plates and replaced with drug or vehicle containing the appropriate concentration. Each concentration was tested in 8 replicas and the results were confirmed in at least three independent experiments.
Luciferase reporter assays
PPARγ transactivation assays
pcDNA3, FLAG-pcDNA3-PPARγ and 3XPPRE-LUC plasmids were purchased from Addgene (Cambridge MA). Approximately 60% confluent HepG2 cells were transfected with 3XPPRE-LUC and pcDNA3 or FLAG-pcDNA3-PPARγ plasmids using FuGENE® 6 (Promega). Transfections were performed in six-well culture plates. Each well had approximately 10-cm2 surface for cell culture growth, and cells in each well were transfected with 2 µg of total plasmid DNA: 1500 ng of 3XPPRE-LUC and 500 ng of pcDNA3 or FLAG-pcDNA3-PPARγ. After 24 hour transfection in the growth media, 10,000 cells were plated in each well of a 96-well culture plate (PerkinElmer) and treated with drugs at the indicated concentrations for an additional 24 h in the assay media. Firefly luciferase activity was measured 48 h after transfection using the Neolite Reporter Gene Assay System (PerkinElmer) and FLUOstar OPTIMA microplate reader (BMG Labtech, Stafford, TX).
NF-κB reporter gene assays
NF-κB reporter stable cells were established by transducing LNCaP cells with lentiviral constructs containing NF-B transcriptional response elements (TREs) linked to the luciferase gene (Cignal™ Lenti Reporter, Qiagen®, Valencia, CA) according to the manufacture’s recommendations. On the second day after transduction, the medium was changed to regular RPMI medium. On the third day, transduced cells were selected in a medium containing puromycin (2.5 µg/ml), a concentration established to kill 100% of the control cells within 3-4 days. To minimize any insertion site bias, pooled populations of transduced cells were used for the assays. Cells were treated with TNFα alone or in combination with SDA. Firefly luciferase activity was measured using the Neolite Luciferase Assay System.
Immunoflourescence (IF)
Cells were grown on Lab-Tek II chamber 8-well glass slides (VWR, Suwanee, GA) for 24 h at 3 x105 cells per well. Appropriate treatments were added as described in the Figure 1 legends. The next day, cells were rinsed with phosphate buffered saline (PBS) and fixed in buffered 4% paraformaldehyde (30 min). Following fixation, cells were rinsed in PBS and treated with absolute methanol for 10 min. Following equilibration in PBS, the cells were incubated in blocking solution (5% normal goat serum, 2% BSA in PBS, pH 7.3) for 10 min then incubated with primary antibody appropriately diluted (1/200 for AR and 1/400 for PPARγ) in blocking solution overnight at room temperature. In each experiment, one well for each treatment group and cell line received no primary antibody to detect non-specific secondary antibody binding. Next day, the cells were washed and treated with secondary antibody in blocker at room temperature for one hour. Slides were mounted with Vectashield (Vector) mounting medium and sealed with clear nail polish and examined with a Nikon Eclipse C1 2000-E confocal microscope. Z-stacks were acquired and rendered with Nikon EZ-C1 3.91 software. To facilitate signal intensity comparisons between treatment groups, images were acquired and processed as follows: The gain for each laser was adjusted to the positive control treatment well, which was scanned first. That value was then used for each z-stack acquisition for each treatment. The control well z-stack was rendered and the saturation and dark levels were adjusted until a good quality image was obtained. Those values were used for subsequently rendered images of each treatment group.
Real-time PCR
Total RNA was isolated using Trizol Reagent Protocol (Life Sciences) according to the manufacturer’s protocol. AR and PPARγ mRNA were amplified with validated human-specific primers according to the Qiagen SABiosciences protocol [38]. PCR products were verified by melting curves using MyiQ PCR cycler (Bio-Rad) and 2% agarose gel electrophoresis.
Western blotting
The effects of SDA and DOX on AR and PPARγ receptor proteins were determined by WB technique as previously described by our laboratory [38]. Briefly, cells were grown in 6-well plates and treated with appropriate receptor agonists (T for AR or ROSI for PPARγ), DOX and SDA alone or in combination for 48 h. Cells were homogenized in RIPA lysis buffer (Sigma) containing protease inhibitors (Sigma). The cell homogenates were centrifuged at 15000 X g for 15 min to remove cellular debris. Protein concentration was measured using the Bio-Rad protein assay. Protein aliquots (20 µg) in Laemmli sample buffer were resolved on precast 10% tris-HCl mini acrylamide gels and electrotransferred to nitrocellulose membranes (Bio-Rad). Membranes were blocked and subsequently incubated with primary antibodies (Santa Cruz Biotechnology) diluted in blocker. Dilutions used were: 1:200 for AR antibody (N-20, sc-816), 1/400 for mouse monoclonal PPARγ (E-8, sc-7273), and 1/1000 for cyclophilin antibody (sc-133494). Blots with primary antibodies were placed on a shaker and incubated overnight. The next day, blots were washed to remove unbound antibodies before incubation with the appropriate horseradish peroxidase (HRP-conjugated) anti-rabbit (sc-2004) or anti-mouse (sc-2005) secondary antibodies (Santa Cruz Biotechnology) for 90 min at 1/1000 dilutions. Membranes were then washed and incubated with chemiluminescent developing reagent (E2400, Denville) for one min and subsequently exposed to x-ray film (E-3012; Denville). The appropriate proteins were visualized by developing the film. The levels of AR and PPARγ proteins were quantified using the Epson 4180 Perfection scanning software (Epson-America). Relative protein amounts (from two experiments) were determined relative to cyclophilin housekeeping gene using the Un-Scan-It software (Silk Scientific, Inc., Orem, UT, USA).
Data analyses
Data from cell culture MTT cell viability and luciferase assays were expressed as the mean ± SD. The data from the transfection assays were analyzed by one-way ANOVA followed by Dunnett’s post hoc test (Graph Pad Prism 5.0, San Diego, CA). P<0.05 was considered statistically significant. MTT data were analyzed by GraphPad Prism® to produce dose-response curves for SDA by using nonlinear analysis. CI values were determined by the third generation "CompuSyn" software written by Nick Mart of MIT using the median-effect method [39]. The CI index was calculated using the formula:
CI = | C A, X | + | C B, X |
IC X, A | IC X, B |
Where C A, X and C B, X are the concentrations of DOX and SDA used in combination to achieve X% drug effect. The IC X, A and IC X, B are the concentrations for single drugs (DOX or SDA) that achieve the same effect. Synergy is defined as CI < 1, additivity is when the CI = 1, and antagonism is when the CI > 1.
To calculate drug effect, the mean OD values for each treatment concentration was subtracted from the mean OD values of cells treated with vehicle and the resulted fractions (between 0-100%) were plotted against drug concentrations in logarithmic scales. Real-time PCR data were analyzed using a modification of the delta delta CT method (ΔΔCT) and data were expressed as fold change relative to positive controls [40].
ResultsTop
Single drug treatments: SDA or DOX treatment of LNCaP, PC3 and DU145 human prostate cancer cell lines inhibited cell proliferation with variable median effect (IC50) values
We determined separate IC50 values for SDA and DOX by measuring cell viability/proliferation rates in LNCaP, PC3 and DU145 cells using the MTT assay (Figure 1). SDA showed IC50 of 116, 145, and 601 µM in PC3, DU145, and LNCaP, respectively (Table 1). Inhibition of cell viability/proliferation by SDA was greater in androgen-independent PC3 and DU145 compared with androgen-dependent LNCaP. Log dose-response curves showed that cell viability decreased significantly at SDA concentrations between 116-145 µM in PC3 and DU145 compared to 600 µM in LNCaP. No significant changes in cell viability were observed in RWPE-1 normal human prostate epithelial cells when treated with SDA or combination of SDA and DOX under similar conditions (Figure 1). The DOX IC50 values were 363, 761, and 802 nM in DU145, PC3, and LNCaP, respectively (Figure 2). Those values (nanomolar range) were significantly lower than those of SDA (micromolar range) in all three cell lines.
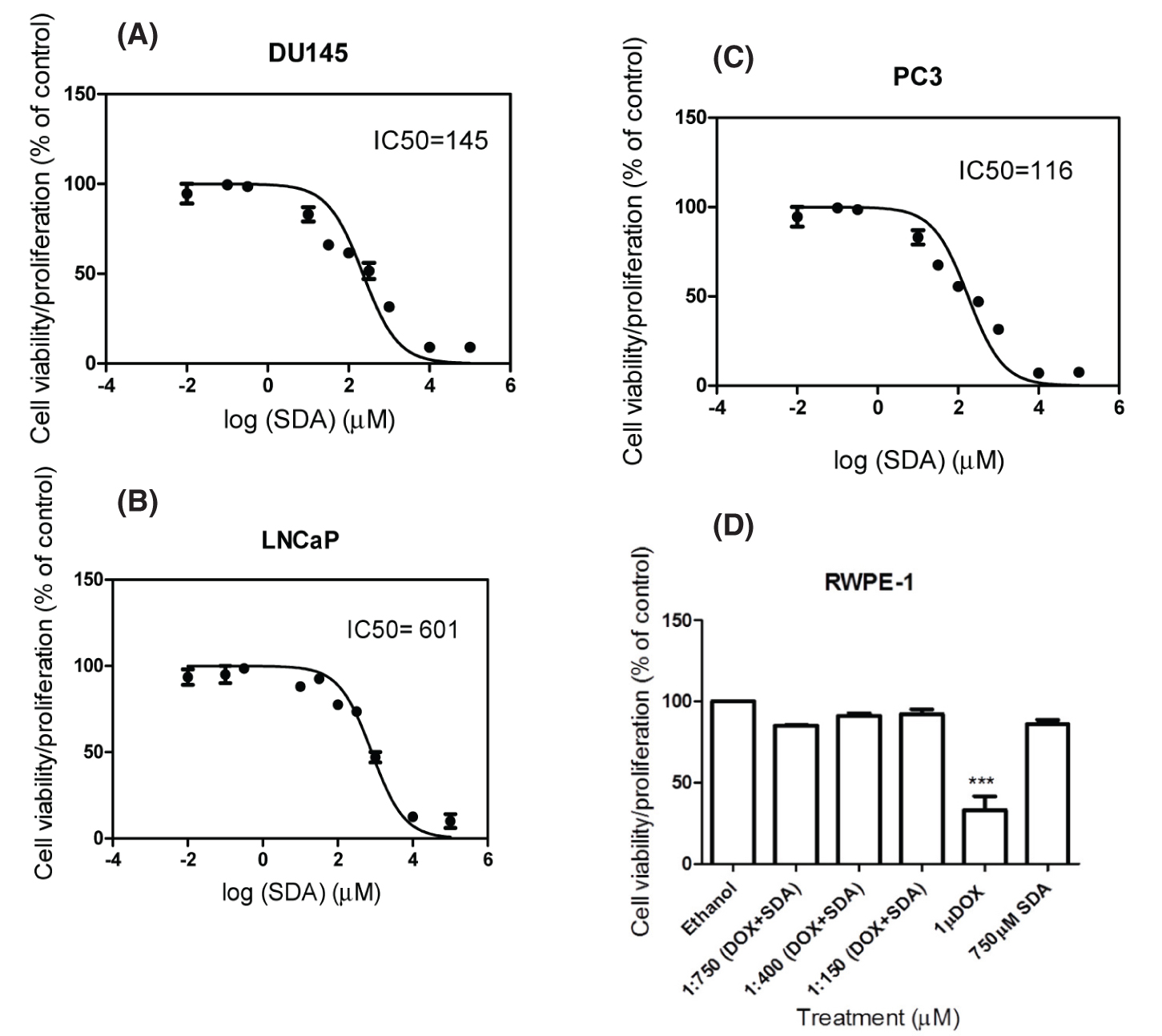
Figure 1 (A-D) Stearidonic acid exerts antitumor activity in human prostate cancer cell lines but has no significant effect on normal prostate epithelial cells when used alone or when mixed with DOX using constant combination ratios derived from IC50 of SDA and DOX in LNCaP (1:750), DU145 (1:400), and PC3 (1:150) (Table 1). (A-C) SDA log-dose response analysis (IC50) in androgen-dependent LNCaP and androgen-independent PC3 and DU145 prostate cancer cells. (D) Treatment of RWPE-1 normal prostate epithelial cells with SDA or SDA=DOX in 1:750, 1:400 and 1:150 constant ratios does not affect cell viability. DOX alone at 1 µM caused significant cell death.
Abbreviations: SDA=stearidonic acid. IC50=SDA concentration (µM) that inhibit 50% of cell viability. Cells were treated for 24-48 h.
Cell line | DOX IC50 (µM) | SDA IC50 (µM) | Ratio used in combo |
LNCaP | 0.802 ±0.1 | 601±10 | (1:750) |
PC3 | 0.760±0.08 | 116±6 | (1:150) |
DU145 | 0.363±0.01 | 145±3 | (1:400) |
Abbreviations: IC50 = single drug concentration that inhibits 50% of cell proliferation; SDA = stearidonic acid; DOX = doxorubicin.
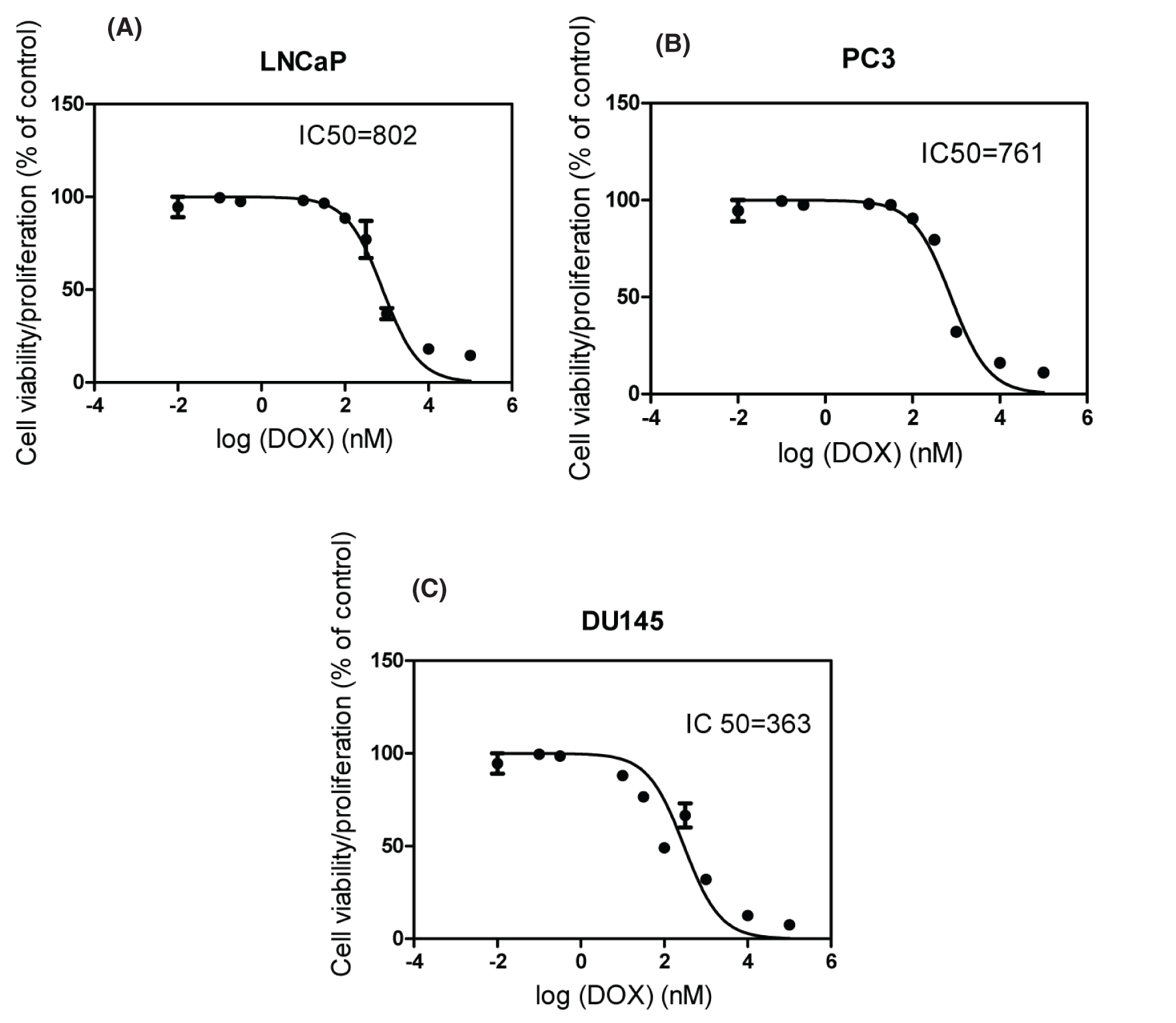
Figure 2 A,B,C Doxorubicin IC50 in human prostate cell cancers. Effect of DOX on cell viability/proliferation in androgen-dependent LNCaP and androgen-independent PC3 cells and DU145 human prostate cancer cell lines using log-dose response analysis.
Abbreviations: DOX=doxorubicin. IC50= DOX concentration that inhibits 50% of cell proliferation. Cells were treated for 24 h.
The cytotoxic effect of DOX is synergistically enhanced by SDA when the two are administered to PC3 and LNCaP cancer cells
To determine if SDA augments DOX-induced cell death we investigated the effect of SDA and DOX alone and in combination on prostate cancer cell death using MTT assay. Results showed that the cytotoxic effect of DOX is synergistically enhanced by SDA when the two are administered to androgen-independent PC3 and androgen-dependent LNCaP cancer cells (Figure 3). The effective dose of the SDA used in combination with DOX was 12x less than its IC50 in LNCaP and 2x less than the its IC50 in PC3.
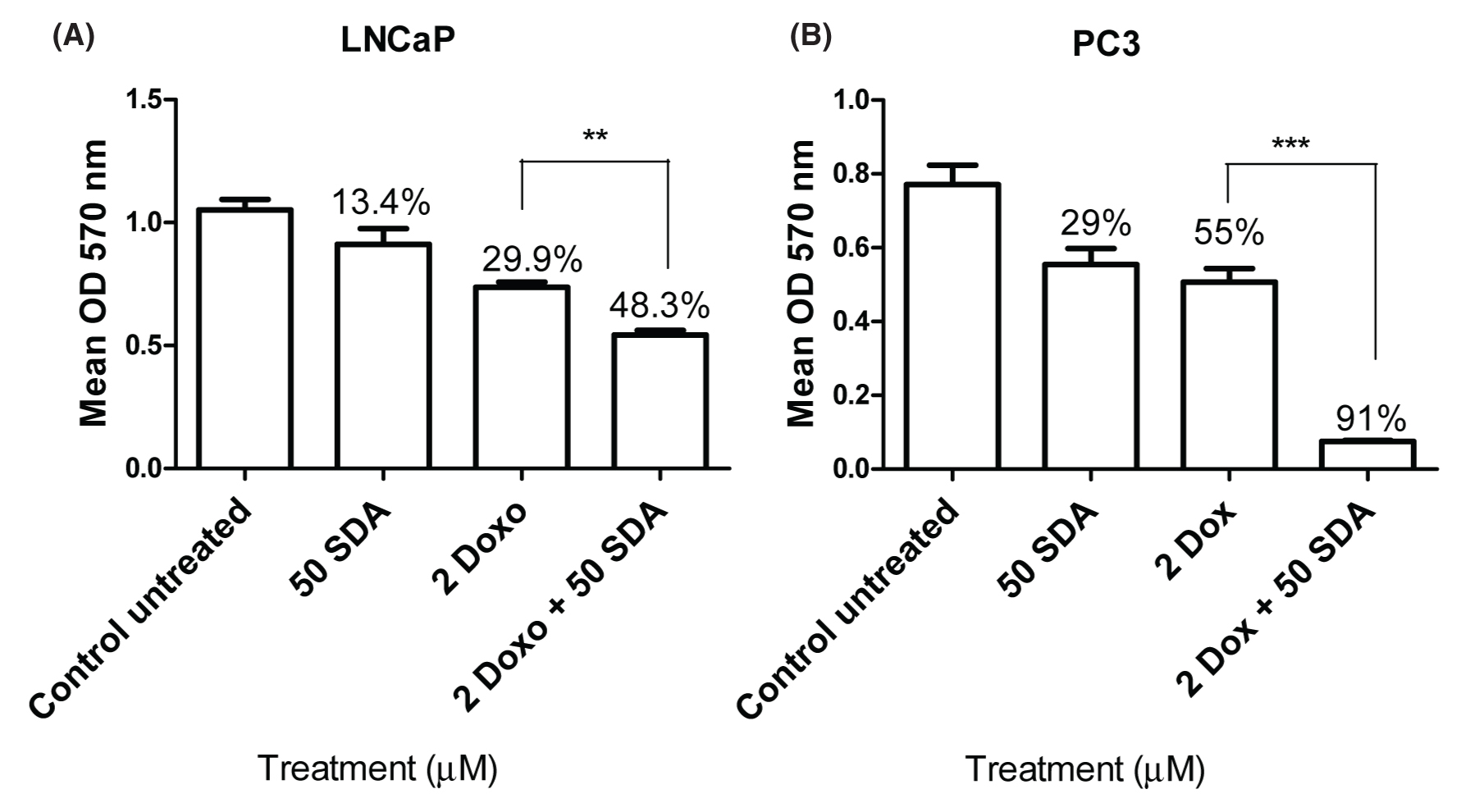
Figure 3 The cytotoxic effect of DOX is synergistically enhanced by SDA when the two are administered to PC3 (A) and LNCaP (B) cancer cells at a constant ratio of 1 (DOX):25 (SDA). SDA/DOX combination is more effective in PC3 than LNCaP with a corresponding reduction in cell viability of 91% in PC3 vs 48.3% in LNCaP when compared to control untreated. Cells were treated for 48 h.
Abbreviations: SDA=stearidonic acid, DOX=doxorubicin, ** (P<0.01), *** (p<0.001)..
SDA inhibited activation/induction of NF-κB in response to the proinflammatory cytokine tumor necrosis factor-alpha (TNFα) in LNCaP cells
Down-regulation of NF-κB is considered critical in halting prostate cancer progression [41]. We therefore investigated the effect of SDA on TNFα-induced NF-κB production in LNCaP cells that were stably transduced with NF-κB reporter gene.
The cells were treated with 50 ng/ml TNFα and 100 µM SDA (about 6 times less than it’s IC50 of 600 µM in LNCaP cells). Treatment with SDA decreased TNFαal-induced NF-κB activation of the promoter activity (Figure 4). At 100 µM, SDA treatment produced about 50% reduction in TNFα-induced NF-κB activity. SDA inhibition of TNFα-induced NF-κB activation was not associated with cytotoxicity (data not shown).
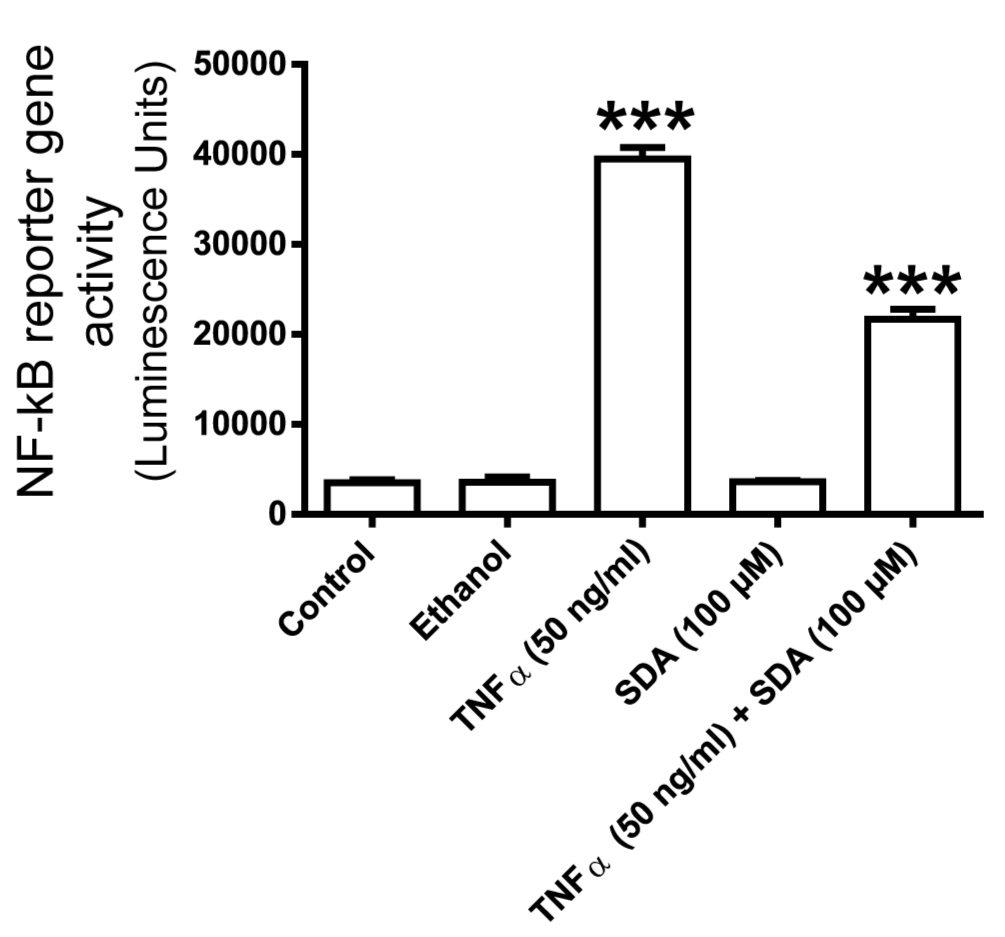
Figure 4 SDA inhibited activation/induction of NF-κB in response to the proinflammatory cytokine tumor necrosis factor-alpha (TNFα) in LNCaP cells. *** (p<0.001). The cells were untreated (control) or treated with ethanol (vehicle for SDA), TNFα or TNFα + SDA as indicated. Twelve-hours after the treatments, NF-κB activated reporter gene expression was determined by measuring the firefly luciferase activity as described in methods. *** (p<0.001).
Drug combinations: DOX and SDA combination induced synergistic antitumor action in human prostate cancer cell lines
We used the method of constant ratio drug combination proposed by Chou and Talalay [39] to determine synergy, additivity, or antagonism of DOX/SDA combination in androgen-dependent LNCaP cells and in the androgen-independent PC3 and DU145 cells. Ratios of DOX to SDA were 1:750 in LNCaP, 1:150 in PC3, and 1:400 in DU145, which were calculated based on the IC50 of DOX and SDA in each cell line (Table 1).
Combination index (CI) analyses of DOX and SDA were performed by the median-effect method using the CompuSyn software and were based on the CI equation described in the materials and methods. Figure 5 illustrates CI values below, at, and above the 1 additivity line. Nonlinear regression analysis showed CI values less than 1 in nearly all treatment cases indicating synergism between SDA and DOX at various combination ratios and effect levels (Table 2). One datum in each of the PC3 and DU145 groups were above 1 indicating antagonism at these particular combined concentrations (Figure 5). Further, in the DU145 cells one datum was equal to 1, which indicates an additive effect. The shapes of the curves (m values) were flat sigmoidal, as the slopes of the median-effect plots were less than 1, in all of our analyses. The CI values obtained at 50-95% drug effect levels were similar in all the cell lines despite the differences in drug ratios (Table 2). Isobologram analysis (a graph that indicates equipotent combinations of various doses of two drugs) for DOX/SDA combinations showed synergism (data not shown).
Cell line | Combination Ratio | Combination index (CI) at effect level % of | Interpretation | |||
ED 50 | ED75 | ED90 | ED95 | |||
LNCaP | (1:750) | 0.47±0.09 | 0.35±0.1 | 0.28±0.1 | 0.24±0.1 | Synergy |
PC3 | (1:150) | 0.5±0.01 | 0.36±0.04 | 0.26±0.09 | 0.22±0.02 | Synergy |
DU145 | (1:400) | 0.38±0.01 | 0.29±0.1 | 0.22±0.1 | 0.18±0.08 | Synergy |
Abbreviations: SDA = stearidonic acid; DOX = doxorubicin
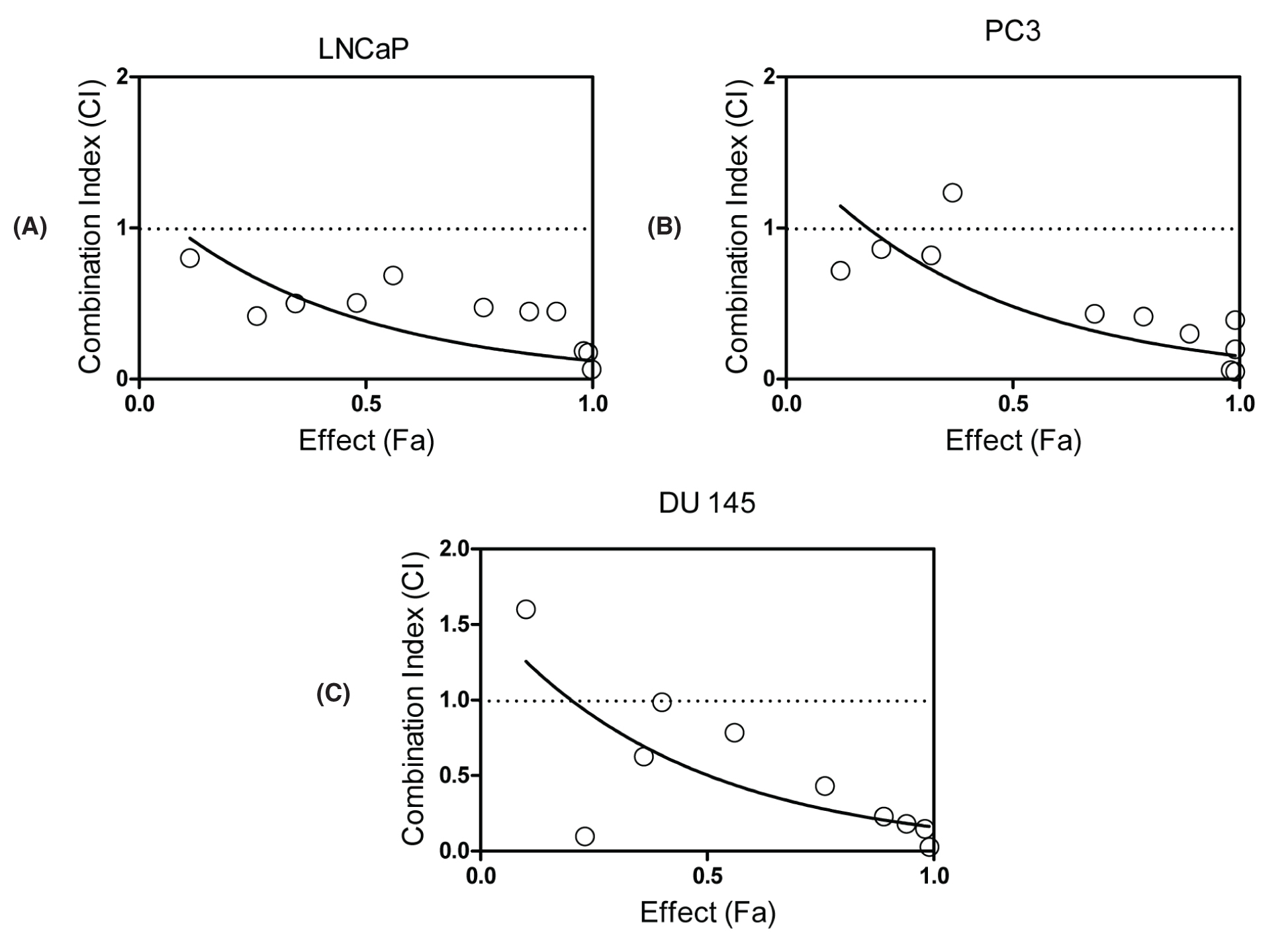
Figure 5 CI curve analysis (Chou and Talalay plot or Fa blot) for DOX/SDA in androgen-dependent LNCaP, and androgen-independent PC3 and DU145 cell lines indicates synergy. The dotted horizontal line at 1 indicates the line of additive effect. CI value less than, equal to, or greater than 1 indicates synergy, additivity, or antagonism, respectively. Effect (Fa) indicates the fractional inhibition for each combinational index. To calculate SDA/DOX concentration for each Fa point the SDA/DOX were mixed using constant ratios corresponding to 0.03, 0.06, 0.12, 0.25, 0.5, 1, 2, 4, 8, 16, 32 times the IC50 for each drug in each cell line. The IC50 for each SDA/DOX are listed in Table 1. Cells were treated for 48 h.
Abbreviations: SDA=stearidonic acid; DOX=doxorubicin.
DOX and SDA inhibited agonist-activated PPARγ in HepG2 cells
To determine any effect of DOX and SDA in modulating PPARγ, a nuclear transcription factor involved in controlling inflammatory genes and lipid metabolism [42, 43], we measured PPARγ transactivation in HepG2 cells transiently transfected with a human PPARγ and 3XPPRE-LUC plasmids. We used HepG2, a human hepatocarcinoma cell line, because it is amenable to transfection with these plasmids [37] whereas, in our hands, all the three prostate cancer cell lines were refractory to transient transfections with these plasmids. Transfected HepG2 cells were treated with rosiglitazone (ROSI) or troglitazone (TRO), synthetic thiazolidinediones (TZDs) agonists of PPARγ, as positive controls for 15 h. Treatment with either of the TZDs (10 µM) induced PPARγ transactivation (Figure 6). To test SDA or DOX effects on PPARγ transactivation, SDA (100 µM) or DOX (1 µM) was included with the TZD treatment. At these concentrations, both SDA and DOX inhibited TZDs-induced PPAR transactivation (Figure 6). Neither SDA nor DOX alone or in combination with TZDs exerted a noticeable cytotoxicity (data not shown).
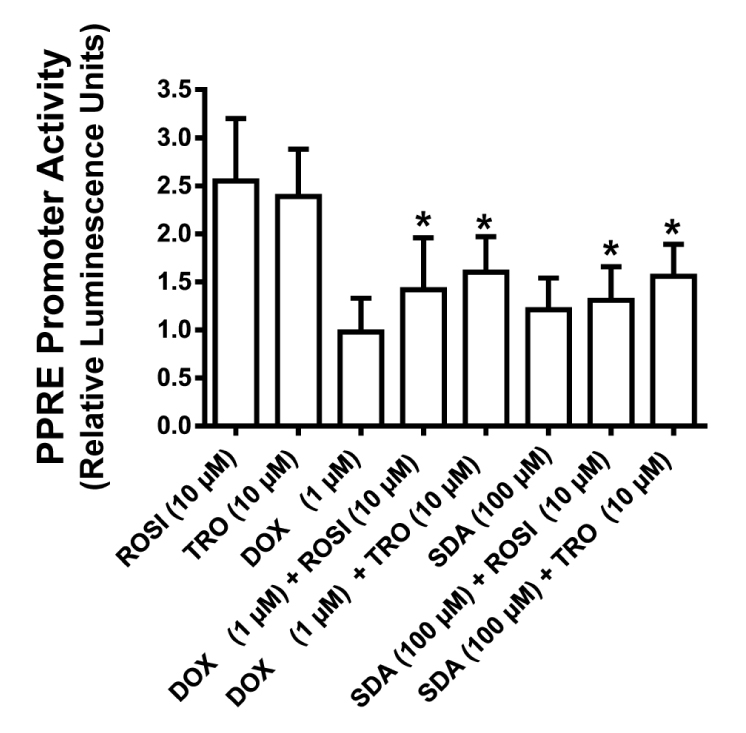
Figure 6 DOX or SDA inhibited ROSI or TRO induced PPARγ transactivation in HepG2 cells. The cells were transiently cotransfected with 3XPPRE-LUC and FLAG-pcDNA3-PPARγ plasmids. After 24 h transfection, the cells were treated with DMSO, ROSI ± DOX, ROSI ± SDA, TRO ± DOX or TRO ± SDA as indicated for another 15 h. The promoter activity was determined by measuring the firefly luciferase activity 15 h after the treatments. The promoter activity is expressed as relative luminescence units and presented as fold change over the DMSO control. Abbreviations: * = (p < 0.05); Tro = Troglitazone.
Treatment with SDA, DOX, or combined DOX/SDA inhibited agonist-induced AR and PPARγ expression in LNCaP and PC3 cells, respectively
Activation of the AR pathway mediates cancer cell growth in CRPC and its inactivation impairs prostate cancer progression [44]. In contrast, the effect of PPARγ varies with the ligand and cancer cells involved [45]. For example, synthetic PPARγ agonists inhibited proliferation and modulated secretory-associated genes in primary culture [46] and in prostate cancer cell lines [47, 48]. We used IF to qualitatively assess the effects of SDA and DOX, alone or in combination, on AR and PPARγ regulation in LNCaP and PC3 cells. Each of the three treatments down regulated T-induced AR fluorescence signal when compared to cells treated with T alone (Figure 7A). Similarly, SDA and DOX treatment, alone or in combination, down regulated PPARγ signal in PC3 cells when compared to Rosi treated positive controls (Figure 7B).
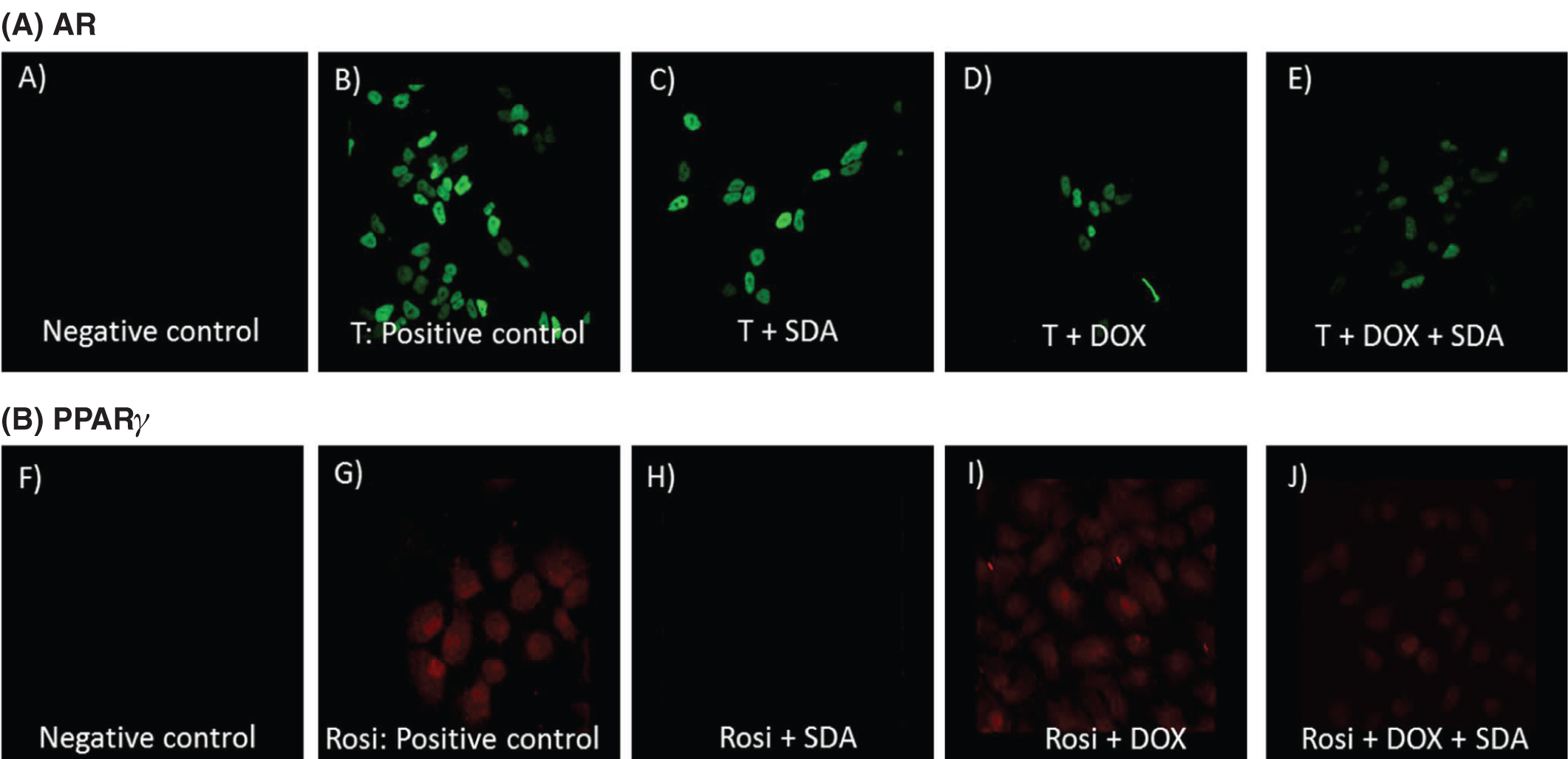
Figure 7 A,B Fluorescence micrographs of LNCaP (A) and PC3 (B) treated with drugs and stained for AR and PPARγ, respectively. Treatment with SDA, DOX, or combined DOX/SDA inhibited agonist-induced AR (A) and PPARγ (B) expression in LNCaP and PC3 cells, respectively. SDA, DOX and DOX + SDA inhibited T-activated AR in androgen-dependent LNCaP cells (A-E). The same treatment inhibited Rosi-induced PPARγ upregulation in androgen-independent PC3 cells (F-J) (see Figures 8 and 9 for quantitative analysis). Treatments of LNCaP cells: 600 µM (SDA) and 0.8 µM (DOX). Treatments of PC3 cells: 116 µM (SDA), 0.76 µM (DOX), and 10 nM (T) for 24 h. The negative control represents non-treated cells or non- stained cells (omission of primary antibody).The apparent signal in panels I and J is DOX autofluorescence [65] and results from accumulation in the cell nuclei by passive diffusion where it intercalates in the DNA.
Abbreviations: T=testosterone; SDA=stearidonic acid; DOX=doxorubicin; AR=androgen receptor; PPARγ = peroxisome proliferator-activated receptor gamma; Rosi=rosiglitazone; T=testosterone; SDA=stearidonic acid; DOX=doxorubicin.
To corroborate the ICC qualitative data, we used real-time PCR and western blotting to quantify the effect of SDA and/or DOX on AR and PPAR mRNA and protein expression levels in LNCaP and PC3 cells. In real-time PCR, treatment of LNCaP cells with DOX and/or SDA inhibited T-induced AR mRNA expression by several fold (Figure 8A). Likewise, treatment of PC3 cells with DOX and/or SDA inhibited Rosi-induced PPAR mRNA when compared with Rosi treated cells alone (Figure 8B).
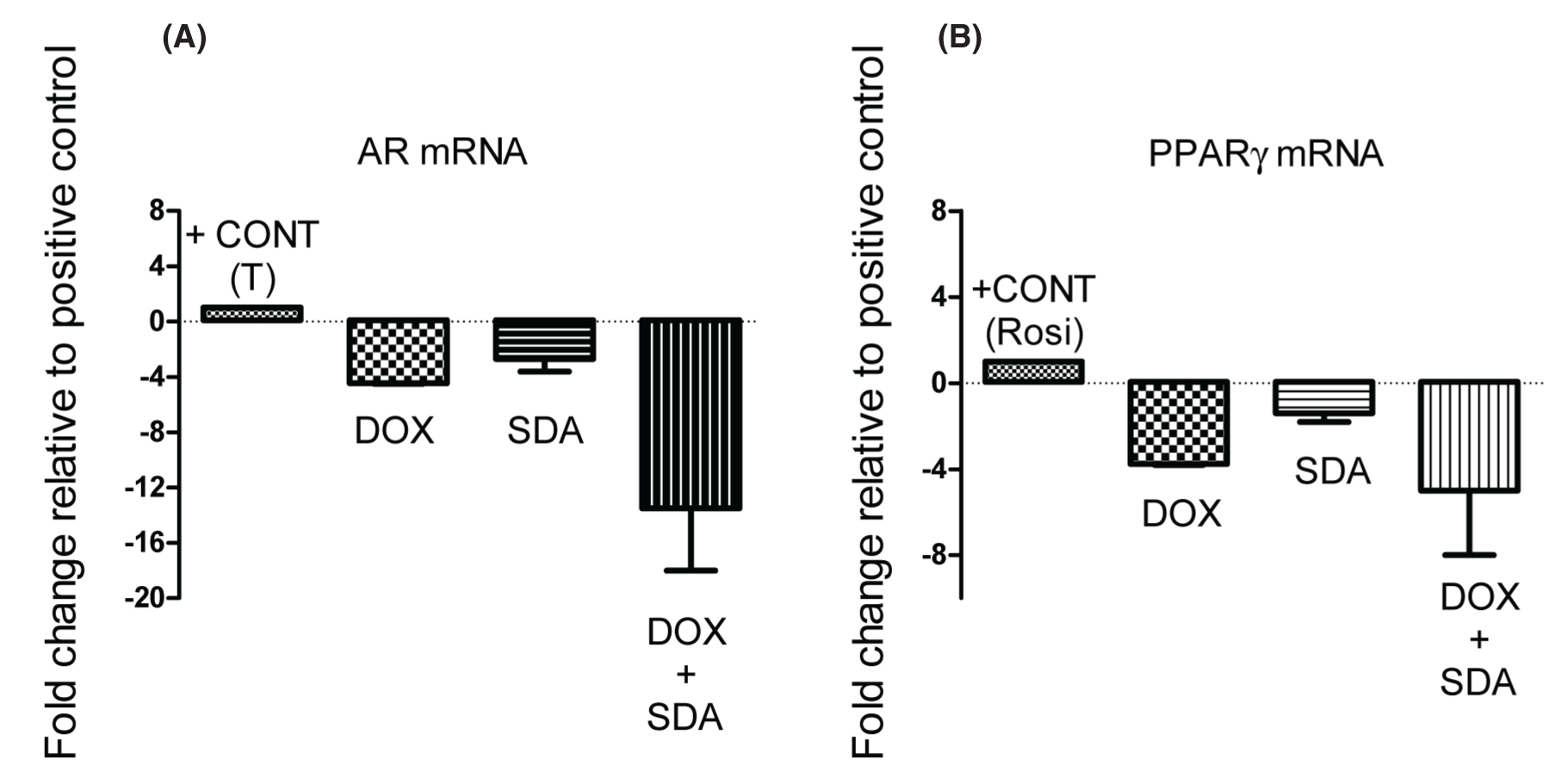
Figure 8 A,B DOX, SDA and SDA + DOX inhibited T-activated AR mRNA expression in androgen-dependent LNCaP cells (A); and Rosi-induced PPARγ mRNA in androgen-independent PC3 cells. Treatments: LNCaP: 600 µM (SDA) and 0.8 µM (DOX). PC3: 116 µM (SDA) and 0.76 µM (DOX). Cells were treated for 48 h.
Abbreviations: T=testosterone; SDA=stearidonic acid; DOX=doxorubicin; Rosi=rosiglitazone; AR=androgen receptor; PPARγ =peroxisome proliferator-activated receptor gamma.
Similar to the real-time PCR data, combination of SDA and DOX, down regulated T-induced AR protein in LNCaP cells when compared to single treatment with DOX or SDA and to T-treated positive controls. Likewise, Rosi-induced PPARγ protein was significantly reduced when PC3 were treated with SDA/DOX combination compared to single treatment with DOX or SDA and to Rosi-treated positive controls (Figure 9).
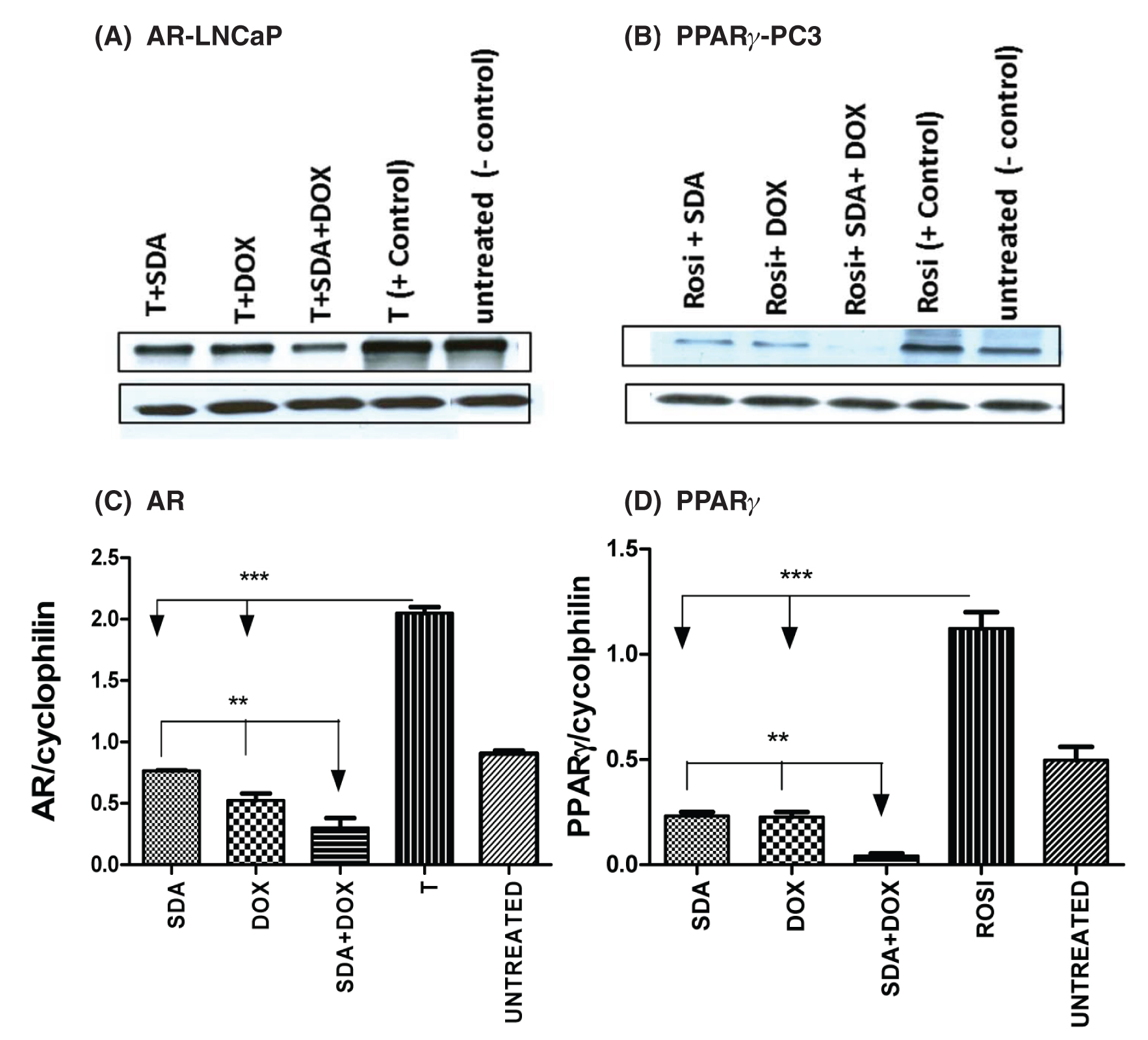
Figure 9 A,B,C,D DOX, SDA and SDA + DOX inhibited T-activated AR protein expression in androgen-dependent LNCaP cells (A); and Rosi-induced PPARγ protein in androgen-independent PC3 cells. Treatments: LNCaP: 600µM (SDA), 0.8 µM (DOX) and 10 nM (T). and PC3: 116 µM (SDA), 0.76 µM (DOX) and 10 µM (ROSI). The western blots are representative of two independent experiments with similar results. Cells were treated for 48 h.
Abbreviations: T=testosterone; SDA=stearidonic acid; DOX=doxorubicin; Rosi=rosiglitazone; AR=androgen receptor; PPARγ =peroxisome proliferator-activated receptor gamma.
DiscussionTop
We tested the antitumor efficacy of the plant-based omega-3 fatty acid SDA and whether it potentiates the antitumor action of DOX in LNCaP, PC3, and DU145, three well-characterized prostate cancer human cell lines. We also investigated the modulatory effects of SDA and DOX, alone and in combinations on AR, NF-κB, and PPARγ, three cancer-related nuclear transcription factors. In single drug treatments, SDA or DOX inhibited cell proliferation in the androgen-dependent LNCaP cells and androgen-independent PC3 and DU145 cells with different IC50s depending on the cell line. Using DOX/SDA combinations, CI analyses showed strong synergism of a DOX/SDA combination in reducing prostate cancer cell viability in concentration ranges significantly lower than when each drug is used alone.
SDA/DOX combination in LNCaP and PC3 were more effective in reducing prostate cancer cell viability than when each drug is used alone. In PC3, SDA/DOX combination caused a reduction in cell viability equivalent to 91% vs 25% reduction by SDA and 55% by DOX when each is used alone. Likewise, SDA/DOX combination causes 48.3% reduction in LNCaP cell viability compared to 13.4% reduction by SDA and 29.9% by DOX. Further, SDA/DOX combination, when used in concentrations equivalent to their 1C50s, decreased agonist-induced expression of nuclear factors AR, PPARγ and NF-κB mRNA and protein. The reason why the combination of SDA and DOX was more effective in reducing cell viability in androgen-refractory prostate cancer cells DU145 and PC3 in comparison to LNCaP cells could be due to the significantly high constitutive activity and expression of NF-κB in PC3 and DU145 compared to LNCaP [49].
We used nonlinear regression methodology to derive IC50 and CI for DOX/SDA combinations. This approach was shown to consistently increase the accuracy of the regression-derived IC50s and CIs when compared to linear methods. Linear regression analysis could lead to underestimation of the concentration effect curves (Fa plot) by producing curves that are shallower than the actual curves thereby leading to underestimation of the effective drug concentration at a wider effect levels ranging from 10 to 80% [50]. Further, we increased the number of replicates (8 per each concentration) as this was also shown to reduce data variability at the extreme ends (<10% and >90% effect levels) of the concentration effect curve [50].
It is worth noting that SDA induces significant reduction of TNFα-induced NF-κB activity in LNCaP cells stably transduced with NF-κB reporter gene. This action mimics that of DHA’s selective modulation of NF-κB in human prostate cancer cells [51]. We considered the SDA-inhibitory effects of NF-κB as a significant finding, especially when viewed in context of the SDA potential synergistic antitumor efficacy with DOX. The SDA-induced suppression of NF-κB can be parsed as significant based on several aspects: First, high levels of NF-κB are considered critical in promoting cell survival and proliferation in human prostate carcinoma cells, especially androgen-independent PC3 and DU145 cells [49, [52]]. Second, the lower unsaturation index of SDA enhances its stability and shelf-life with a simultaneous reduction in off flavors and odors when compared with EPA and DHA from fish oil [24]. Third, SDA as a nutrient bypasses the rate-limiting step delta-6-desaturase in the conversion of ALA to EPA and DHA, a highly inefficient enzymatic process in humans. Fourth, undesirable NF-κB activity is induced in response to chemotherapy [53, 54] and therefore combining SDA with DOX could down regulate the NF-κB activity that promotes PCa cell growth and increases resistance to chemotherapy.
Similar to the inhibitory effect of SDA on NF-κB, both SDA and DOX suppressed TZDs-induced transactivation of PPARγ in HepG2 cells transiently transfected with human PPARγ and 3XPPRE-LUC plasmids. In addition, SDA and/or DOX blunted T-induced AR and TZD-induced PPARγ proteins in IF and western blotting studies. Similar results were shown by real-time PCR. In IF studies the fluorescence intensity of the two proteins were either unchanged or down-regulated when compared to cells treated with positive controls.
Our results suggest that the antitumor activity of SDA in prostate cancer cells is mediated, at least in part, via repression of more than one transcription factor including NF-κB, PPARγ and AR. The SDA inhibition of NF-κB and PPARγ corroborates similar findings in MDA-MB-231 breast cancer cells where SDA treatment was more effective than α-linolenic acid (LNA) in reducing Cox-2, NF-κB, and PPARγ mRNA expression when measured by real-time PCR. Further, the down-regulation of AR by SDA mimics the suppression of AR implicated in inhibition of prostate cancer progression to a CRPC state when treated by the marine-based EPA and DHA [33].
The blocking of TZDs-induced PPARγ transactivation by SDA in our study contrasts with the reported up-regulatory effects of EPA and DHA on this nuclear receptor [43, 55, 56]. This suggests that the antitumor action of SDA may involve inhibition of PPARγ whereas that of omega-3 (n-3) EPA and DHA polyunsaturated fatty acids (PUFAs) involves activation of PPARγ and related PPARγ target genes leading to ultimate reduction in inflammatory responses [55]. The reason why SDA-induced inhibition of PPARγ is beneficial is not clear and requires further analysis in future studies.
Studies suggest that EPA and DHA omega-3 fatty acids down regulate NF-κB through decreased production of cytokines such as TNFα [34]. The SDA suppression of TNFα-induced NF-κB signaling in our study mimics this latter mechanism. The inhibitory effects of DHA and EPA are thought to decrease IκBα phosphorylation and subsequently suppress the activation of NF-κB. In prostate cancer, downstream effects of NF-κB suppression include lowering of angiogenesis, metastasis, and cellular invasion.
Similar to SDA, DOX blunted the transactivation of PPARγ by TZDs PPARγ full agonists. Although there are multiple mechanisms by which DOX inhibits the growth of cancer cells in general [57, 58], inhibition of PPARγ by DOX in HepG2 cells transfected with PPARγ supports the hypothesis that implicates DOX-induced inhibition of PPARγ in type-2-diabetes-like conditions in rodent models [59].
The mechanism by which SDA sensitizes the antitumor activity of DOX could be related to SDA-induced suppression of NF-κB, AR and/or PPARγ. It is well known that inhibition of NF-κB activation sensitizes cancer cells to DOX-induced apoptosis [60, 62]. Involvement of downstream signal transduction factors in SDA-induced inhibition of NF-κB is unknown. It is possible that the inhibition of NF-κB by SDA could be associated with increased activity (decreased phosphorylation) of the NF-κB inhibitory molecule IκBα or through suppression of protein kinase C epsilon (PKCε), an essential mediator of NF-κB activation in PCa cells [63]. Further studies are needed to substantiate these hypotheses.
The lack of antiproliferative activity of SDA on RWPE-1 normal prostate epithelial cells suggests that SDA does not impinge on the phenotype of non-transformed prostate cells and could be used as a safe dietary component to selectively inhibit prostate cancer cell proliferation.
ConclusionTop
Our findings suggest that SDA could be used as a dietary supplement in combination chemotherapy with DOX and possibly with other similar chemotherapeutic drugs. However, the reported cardioprotective effects of SDA [64] makes this supplement an attractive candidate for combination with DOX in future clinical translation studies in prostate cancer.
Acknowledgements
This work was supported by funding through Auburn University Research Initiative in Cancer (AURIC) and Animal Health and Disease Research (AHDR) to M. Mansour as well as Scot-Ritchey Research Center (SRRC) and Animal Health and Disease Research (AHDR) to S. Pondugula.
Conflict of interest
The authors declare no conflict of interest.
ReferencesTop
[1] Crawford ED (2003) Epidemiology of prostate cancer. Urology 62:3–12. Article Pubmed
[2] Damber JE, Aus G (2008) Prostate cancer. Lancet 371:1710–1721. Article Pubmed
[3] Di Lorenzo G, Autorino R, Figg WD, De Placido S (2007) Hormone-refractory prostate cancer: where are we going? Drugs 67:1109–1124. Article Pubmed
[4] Chang SS (2007) Treatment options for hormone-refractory prostate cancer. Rev Urol 9:S13–18. Article Pubmed
[5] Chou TC (2006) Theoretical basis, experimental design, and computerized simulation of synergism and antagonism in drug combination studies. Pharmacol Rev 58:621–681. Article Pubmed
[6] Petrylak DP, Tangen CM, Hussain MH, Lara PN Jr, Jones JA, et al. (2004)Docetaxel and estramustine compared with mitoxantrone and prednisone for advanced refractory prostate cancer. N Engl J Med 351:1513–1520. Article Pubmed
[7] Tannock IF, de Wit R, Berry WR, Horti J, Pluzanska A, et al. (2004) Docetaxel plus prednisone or mitoxantrone plus prednisone for advanced prostate cancer. N Engl J Med 351:1502–1512. Article Pubmed
[8] de Bono JS, Oudard S, Ozguroglu M, Hansen S, Machiels JP, et al. (2010) Prednisone plus cabazitaxel or mitoxantrone for metastatic castration-resistant prostate cancer progressing after docetaxel treatment: a randomised open-label trial. Lancet 376:1147–1154. Article Pubmed
[9] Hardman WE, Moyer MP, Cameron IL (2000) Dietary fish oil sensitizes A549 lung xenografts to doxorubicin chemotherapy. Cancer Lett 151:145–151. Article Pubmed
[10] Hardman WE, Avula CP, Fernandes G, Cameron IL (2001)Three percent dietary fish oil concentrate increased efficacy of doxorubicin against MDA-MB 231 breast cancer xenografts. Clin Cancer Res 7:2041–2049. Article Pubmed
[11] Germain E, Lavandier F, Chajes V, Schubnel V, Bonnet P, et al. (1999) Dietary n-3 polyunsaturated fatty acids and oxidants increase rat mammary tumor sensitivity to epirubicin without change in cardiac toxicity. Lipids 34:S203. Article Pubmed
[12] Shao Y, Pardini L, Pardini RS (1995) Dietary menhaden oil enhances mitomycin C antitumor activity toward human mammary carcinoma MX-1. Lipids 30:1035-1045. Article Pubmed
[13] Cha MC, Meckling KA, Stewart C (2002)Dietary docosahexaenoic acid levels influence the outcome of arabinosylcytosine chemotherapy in L1210 leukemic mice. Nutr Cancer 44:176–181. Article Pubmed
[14] Hardman WE, Moyer MP, Cameron IL (1999) Fish oil supplementation enhanced CPT-11 (irinotecan) efficacy against MCF7 breast carcinoma xenografts and ameliorated intestinal side-effects. Br J Cancer 81:440–448. Article Pubmed
[15] Cortes-Funes H, Coronado C (2007) Role of anthracyclines in the era of targeted therapy. Cardiovasc Toxicol 7:56–60. Article Pubmed
[16] Arcamone F, Cassinelli G, Fantini G, Grein A, Orezzi P, et al. (1969) Adriamycin, 14-hydroxydaunomycin, a new antitumor antibiotic from S. peucetius var. caesius. Biotechnol Bioeng 11:1101–1110. Article Pubmed
[17] Weiss RB (1992) The anthracyclines: will we ever find a better doxorubicin? Semin Oncol 19:670–686. Article Pubmed
[18] Gewirtz DA (1999) A critical evaluation of the mechanisms of action proposed for the antitumor effects of the anthracycline antibiotics adriamycin and daunorubicin. Biochem Pharmacol 57:727–741. Article Pubmed
[19] Tokarska-Schlattner M, Zaugg M, Zuppinger C, Wallimann T, Schlattner U (2006) New insights into doxorubicin-induced cardiotoxicity: The critical role of cellular energetics. J Mol Cell Cardiol 41:389–405. Article Pubmed
[20] Zhang Y, Song S, Yang F, Au JL, Wientjes MG (2001) Nontoxic doses of suramin enhance activity of doxorubicin in prostate tumors. J Pharmacol Exp Ther 299:426–433. Article Pubmed
[21] Tsakalozou E, Eckman AM, Bae Y (2012) Combination effects of docetaxel and Doxorubicin in hormone-refractory prostate cancer cells. Biochem Res Int 2012:832059. Article Pubmed
[22] Das A, Durrant D, Mitchell C, Mayton E, Hoke NN, et al. (2010) Sildenafil increases chemotherapeutic efficacy of doxorubicin in prostate cancer and ameliorates cardiac dysfunction. Proc Natl Acad Sci USA 107:18202–18207. Article Pubmed
[23] Kelavkar UP, Hutzley J, Dhir R, Kim P, Allen KG, et al. (2006) Prostate tumor growth and recurrence can be modulated by the omega-6:omega-3 ratio in diet: athymic mouse xenograft model simulating radical prostatectomy. Neoplasia 8:112–124. Article Pubmed
[24] Whelan J (2009) Dietary Stearidonic Acid Is a Long Chain (n-3) polyunsaturated fatty acid with potential health benefits. J Nutr 139:5–10. a href="http://jn.nutrition.org/content/139/1/5.long">Article Pubmed
[25] Harris WS, Miller M, Tighe AP, Davidson MH, Schaefer EJ (2008) Omega-3 fatty acids and coronary heart disease risk: clinical and mechanistic perspectives. Atherosclerosis 197:12–24. Article Pubmed
[26] von Schacky C, Harris WS (2007) Cardiovascular benefits of omega-3 fatty acids. Cardiovasc Res 73:310–315. Article Pubmed
[27] Harris WS, DiRienzo MA, Sands SA, George C, Jones PG, et al. (2007) Stearidonic acid increases the red blood cell and heart eicosapentaenoic acid content in dogs. Lipids 42:325–333. Article Pubmed
[28] Harris WS (2007) Expert opinion: omega-3 fatty acids and bleeding-cause for concern? Am J Cardiol 99:44C–46C. Article Pubmed
[29] Berquin IM, Edwards IJ, Chen YQ (2008) Multi-targeted therapy of cancer by omega-3 fatty acids. Cancer Lett 269:363–377. Article Pubmed
[30] Siddiqui RA, Shaikh SR, Sech LA, Yount HR, Stillwell W, et al. (2004) Omega 3-Fatty Acids: Health benefits and cellular mechanisms of action. Mini Rev Med Chem 4:859–871. Article Pubmed
[31] Berquin IM, Min Y, Wu R, Wu J, Perry D, et al. (2007) Modulation of prostate cancer genetic risk by omega-3 and omega-6 fatty acids. J Clin Invest 117:1866–1875. Article Pubmed
[32] Guil-Guerrero JL (2007) Stearidonic acid (18:4n-3): Metabolism, nutritional importance, medical uses and natural sources. Eur J Lipid Sci Technol 109:1226–1236. Article
[33] Friedrichs W, Ruparel SB, Marciniak RA, deGraffenried L (2011) Omega-3 fatty acid inhibition of prostate cancer progression to hormone independence is associated with suppression of mTOR signaling and androgen receptor expression. Nutr Cancer 63:771–777. Article Pubmed
[34] Calder PC (2012) Mechanisms of action of (n-3) fatty acids. J Nutr 142:592S–599S. Article Pubmed
[35] David-Beabes GL, Overman MJ, Petrofski JA, Campbell PA, de Marzo AM, et al. (2000) Doxorubicin-resistant variants of human prostate cancer cell lines DU 145, PC-3, PPC-1, and TSU-PR1: characterization of biochemical determinants of antineoplastic drug sensitivity. Int J Oncol 17:1077–1086. Article Pubmed
[36] Shen F, Chu S, Bence AK, Bailey B, Xue X, et al. (2008) Quantitation of doxorubicin uptake, efflux, and modulation of multidrug resistance (MDR) in MDR human cancer cells. J Pharmacol Exp Ther 324:95–102. Article Pubmed
[37] Pondugula SR, Brimer-Cline C, Wu J, Schuetz EG, Tyagi RK, et al. (2009) A phosphomimetic mutation at threonine-57 abolishes transactivation activity and alters nuclear localization pattern of human pregnane x receptor. Drug Metab Dispos 37:719–730. Article Pubmed
[38] Mansour M, Schwartz D, Judd R, Akingbemi B, Braden T, et al. (2011) Thiazolidinediones/PPARgamma agonists and fatty acid synthase inhibitors as an experimental combination therapy for prostate cancer. Int J Oncol 38:537–546. Article Pubmed
[39] Chou TC, Talalay P (1984) Quantitative analysis of dose-effect relationships: the combined effects of multiple drugs or enzyme inhibitors. Adv Enzyme Regul 22:27–55. Article Pubmed
[40] Livak KJ, Schmittgen TD (2001) Analysis of relative gene expression data using real-time quantitative PCR and the 2(-Delta Delta C(T)) Method. Methods 25:402–408. Article Pubmed
[41] Sarkar FH, Li Y, Wang Z, Kong D(2010) Novel targets for prostate cancer chemoprevention. Endocr Relat Cancer 17:R195-212. Article Pubmed
[42] Schmitz G, Ecker J (2008) The opposing effects of n−3 and n−6 fatty acids. Prog Lipid Res 47:147–155. Article Pubmed
[43] Edwards IJ, O'Flaherty JT (2008) Omega-3 Fatty Acids and PPAR gamma in Cancer. PPAR Res 2008:358052. Article Pubmed
[44] Craft N, Shostak Y, Carey M, Sawyers CL (1999) A mechanism for hormone-independent prostate cancer through modulation of androgen receptor signaling by the HER-2/neu tyrosine kinase. Nat Med 5:280–285. Article Pubmed
[45] Krishnan A, Nair SA, Pillai MR (2007) Biology of PPAR gamma in cancer: a critical review on existing lacunae. Curr Mol Med 7:532–540. Article Pubmed
[46] Xu Y, Iyengar S, Roberts RL, Shappell SB, Peehl DM (2003) Primary culture model of peroxisome proliferator-activated receptor gamma activity in prostate cancer cells. J Cell Physiol 196:131–143. Article Pubmed
[47] Ban JO, Oh JH, Son SM, Won D, Song HS, et al. (2011) Troglitazone, a PPAR agonist, inhibits human prostate cancer cell growth through inactivation of NFkappaB via suppression of GSK-3beta expression. Cancer Biol Ther 12:288–296. Article Pubmed
[48] Koeffler HP (2003) Peroxisome Proliferator-activated Receptor and Cancers. Clin Cancer Res 9:1–9. Article Pubmed
[49] Gasparian AV, Yao YJ, Kowalczyk D, Lyakh LA, Karseladze A, et al. (2002) The role of IKK in constitutive activation of NF-kappaB transcription factor in prostate carcinoma cells. J Cell Sci 115(Pt 1):141–151. Article Pubmed
[50] Zhao L, Wientjes MG, Au JL (2004) Evaluation of combination chemotherapy: integration of nonlinear regression, curve shift, isobologram, and combination index analyses. Clin Cancer Res 10:7994–8004. Article Pubmed
[51] Cavazos DA, Price RS, Apte SS, deGraffenried LA (2011) Docosahexaenoic acid selectively induces human prostate cancer cell sensitivity to oxidative stress through modulation of NF-kappaB. Prostate 71:1420–1428. Article Pubmed
[52] Catz SD, Johnson JL (2001) Transcriptional regulation of bcl-2 by nuclear factor kappa B and its significance in prostate cancer. Oncogene 20:7342–7351. Article Pubmed
[53] Cusack JC Jr., Liu R, Houston M, Abendroth K, Elliott PJ, et al. (2001) Enhanced chemosensitivity to CPT-11 with proteasome inhibitor PS-341: implications for systemic nuclear factor-kappaB inhibition. Cancer Res 61:3535–3540. Article Pubmed
[54] Basu S, Rosenzweig KR, Youmell M, Price BD (1998) The DNA-dependent protein kinase participates in the activation of NF kappa B following DNA damage. Biochem Biophys Res Commun 247:79–83. Article Pubmed
[55] Deckelbaum RJ, Worgall TS, Seo T (2006) n−3 Fatty acids and gene expression. The Am J Clin Nutr 83:S1520–1525S. Article Pubmed
[56] Anderson BM, Ma DW (2009) Are all n-3 polyunsaturated fatty acids created equal? Lipids Health Dis 8:33. Article Pubmed
[57] Scherf U, Ross DT, Waltham M, Smith LH, Lee JK, et al. (2000) A gene expression database for the molecular pharmacology of cancer. Nat Genet 24:236–244. Article Pubmed
[58] Jackson TL (2003) Intracellular accumulation and mechanism of action of doxorubicin in a spatio-temporal tumor model. J Theor Biol 220:201–213. Article Pubmed
[59] Arunachalam S, Tirupathi Pichiah PB, Achiraman S (2013) Doxorubicin treatment inhibits PPARγ and may induce lipotoxicity by mimicking a type 2 diabetes-like condition in rodent models. FEBS Lett 587:105–110. Article Pubmed
[60] Arlt A, Vorndamm J, Breitenbroich M, Folsch UR, Kalthoff H, et al. (2001) Inhibition of NF-kappaB sensitizes human pancreatic carcinoma cells to apoptosis induced by etoposide (VP16) or doxorubicin. Oncogene 20:859–868. Article Pubmed
[61] Somerville L, Cory JG (2000) Enhanced roscovitine-induced apoptosis is mediated by a caspase-3-like activity in deoxyadenosine-resistant mouse leukemia L1210 cells. Anticancer Res 20:3347–3355. Pubmed
[62] Manna SK, Aggarwal BB (1999) Lipopolysaccharide inhibits TNF-induced apoptosis: role of nuclear factor-kappaB activation and reactive oxygen intermediates. J Immunol 162:1510–1518. Article Pubmed
[63] Garg R, Blando J, Perez CJ, Wang H, Benavides FJ, et al. (2012) Activation of nuclear factor κB (NF-κB) in prostate cancer is mediated by protein kinase C epsilon (PKCepsilon). J Biol Chem 287:37570–37582. Article Pubmed
[64] Harris WS, Lemke SL, Hansen SN, Goldstein DA, DiRienzo MA, et al. (2008) Stearidonic acid-enriched soybean oil increased the omega-3 index, an emerging cardiovascular risk marker. Lipids 43:805–811. Article Pubmed
[65] Shen F, Chu S, Bence AK, Bailey B, Xue X, et al. (2008) Quantitation of doxorubicin uptake, efflux, and modulation of multidrug resistance (MDR) in MDR human cancer cells. J Pharmacol Exp Ther 324:95–102. Article Pubmed